16 min read • Energy, Utilities & Resources
Decarbonizing industrial heat to face climate change
Is thermal energy storage the missing link in Europe’s industrial decarbonization strategy?
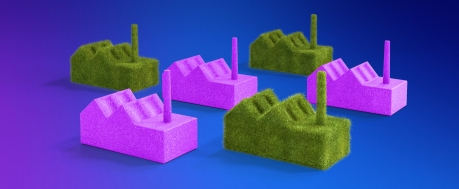
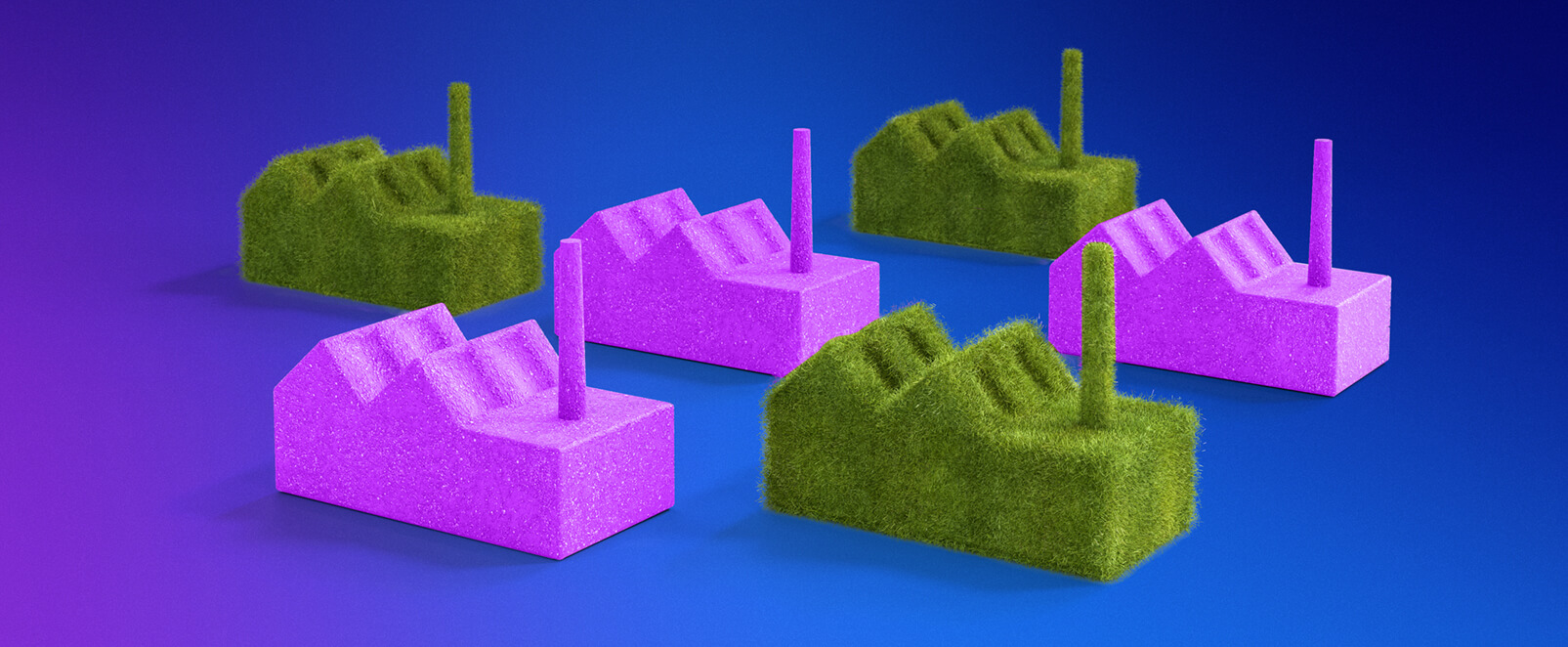
Executive Summary
Decarbonizing industrial heat consumption is crucial to reduce carbon emissions and mitigate the effects of climate change. Currently, the top five heat-demanding industries in the EU — chemical & petrochemical; nonmetallic minerals; food, beverage & tobacco; paper pulp, paper & graphic arts; and iron & steel — account for more than 80% of heat consumption and urgently need decarbonization strategies.
Direct and indirect electrification, carbon capture, utilization, and storage (CCUS), and biomass are among the main technologies for decarbonizing industrial heat. Thermal energy storage (TES) is a potential solution for direct electrification, particularly for industries with process temperatures that reach up to 500°C. Key strengths of TES technology include storage opportunities (i.e., ability to store heat for later use and to integrate non-programmable renewable systems such as photovoltaics and wind), ease of installation, modularity, and cost competitiveness.
Utilities can seize the opportunity and be pioneers in this market by focusing on testing the technology and building partnerships with vendors to speed up commercial deployment. Additionally, they can implement advocacy strategies with European and local authorities.
Innovative solutions like TES can help industrial customers achieve their decarbonization goals, contributing to a low-carbon economy.
1
EUROPEAN INDUSTRIAL HEAT CONSUMPTION
DECARBONIZATION AS A MUST-HAVE
Regulators in Europe are accelerating the transition toward a low-carbon economy, pushing the industrial sector to reduce the CO2 footprint. The EU has set ambitious targets to reduce greenhouse gas emissions by at least 55% by 2030 and achieve carbon neutrality by 2050. However, despite renewable expansion and the increasing attention on the hydrogen economy, these targets are projected to be missed. New strategies are needed to achieve climate targets, and decarbonization of industrial heat consumption through innovative technologies will play a pivotal role.
Industrial heat consumption accounts for a significant portion of global energy usage, with two-thirds of industrial energy demand and almost a fifth of global energy consumption attributed to it, according to the International Energy Agency (IEA). Most industrial heat is generated through the combustion of natural gas, fossil fuels, and petrol, resulting in the emission of substantial amounts of CO2. Thus, decarbonizing industrial heat consumption is essential to reduce carbon emissions.
EUROPEAN ADDRESSABLE MARKET
In Europe, decarbonization of industrial heat consumption offers an opportunity for energy players and vendors to develop innovative solutions to replace non-green fuels and to jump into a market that will face a boost in the coming years.
According to Eurostat, the European industrial sector consumes ~2,500 terawatts per hour (TWh) of energy from different sources (see Figure 1), of which ~1,800 TWh are used to produce heat (accounting for 72% of the market). The share of natural gas, petrol, and fossil fuels used to produce heat is around 60% of the total, generating an addressable market for decarbonization of ~1,100 TWh.
TOP 5 HEAT-DEMANDING INDUSTRIES
Industrial heat consumption is highly concentrated in five industries, which comprise more than 80% of consumption: chemical & petrochemical; nonmetallic minerals; food, beverage & tobacco; paper pulp, paper & graphic arts; and iron & steel. These industries use fuels such as natural gas, fossil fuel, and petrol for heat generation. As Figure 2 illustrates, the heat to be decarbonized often exceeds 60% of the total sources of fuel used for heat generation. Together, these industries show an addressable market (i.e., the total amount of natural gas, fossil fuels, and petrol used to produce heat to be decarbonized) equal to ~930 TWh.
INDUSTRY OPPORTUNITIES
The addressable market of these top five industries can also be examined according to temperature, as shown in Figure 3, revealing substantial differences among them and demonstrating that some industries are more suitable than others for decarbonization (e.g., industries with lower-temperature production processes are significantly more suitable for decarbonization than those using higher temperatures). In temperatures exceeding 500°C, competition of natural gas, fossil fuels, and petrol are very high and electrification technologies are still under development.
Analyzing the production processes of the top five industries in terms of heat demand reveals the following about their decarbonization potential:
-
Chemical & petrochemical — temperatures concentrated in the range of 100°C-500°C. The pharmaceutical subindustry represents a huge opportunity for operators trying to push decarbonization due to its low temperatures (not exceeding 250°C), suitability of the processes, and use of technology that can be easily replaced (e.g., gas boilers, which are used mainly in granulation and melting phases).
-
Nonmetallic minerals — very high temperatures (often more than 1,000°C). Three main subindustries include:
-
Concrete — temperatures reaching 1,500°C (clinker-manufacturing phase), for which furnaces fueled by gas/oil are widespread. However, the use of biomass and hydrogen are gaining attention.
-
Ceramic — temperatures reaching 1,200°C (firing phase) through the use of tunnel kilns fueled by gas/oil. There is a strong interest in hydrogen for this industry. Electrification in the short term could face barriers, but green technologies are possible for the drying phase (up to 200°C).
-
Glass — temperatures ranging from 500°C (surface-heating phase) to 800°C (melting phase), leaving more room for decarbonization compared to concrete and ceramic. This subindustry mainly uses gas ovens. Within the nonmetallic mineral segment, glass may have the greatest potential for decarbonization, as electric ovens already have a high degree of maturity.
-
Food, beverage & tobacco — temperatures mainly below 200°C. In this industry, meat and milk and dairy have the most potential in terms of size and suitability of the processes. Cooking (80°C-120°C) and pasteurization (60°C-70°C) phases can be easily electrified (currently using mainly gas boilers and plate heat exchangers, respectively). Technologies such as heat pumps are already gaining market share.
-
Iron & steel — very high temperatures (mainly more than 1,000°C) for all process phases (i.e., sintering, melting, and rolling). Industry players have widely adopted gas furnaces. However, electrification technologies (e.g., electric arc furnaces) are possible, including hybrid configurations that involve the use of hydrogen. Huge CAPEX, retrofitting costs, and high electricity prices are the main barriers for electrification within the industry. Strong political commitment from regulators in providing incentives and subsidies can be a boost for electrification in the short term.
-
Paper pulp, paper & graphic arts — temperatures mainly below 200°C. Industry is well suited to electrification due to low temperatures in all process phases (i.e., pulping, washing and bleaching, pressing and drying, and calendering) and technologies that exist already in the market. However, this industry is characterized by extensive use of cogenerations fueled by gas, for which major industry players invested a lot of capital. Furthermore, the industry is investigating the use of black liquor (a natural byproduct obtained from paper processing) to feed cogenerations as an alternative to gas and a complementary strategy to decarbonize heat consumption. Electrification is possible in the short to medium term, but considerable barriers (cogenerations) and threats (use of black liquor) exist.
To summarize, utilities should focus first on three main industries to push decarbonization of industrial heat consumption: food, beverage & tobacco; chemical & petrochemical; and paper pulp, paper & graphic arts.
2
DECARBONIZATION STRATEGIES
OPPORTUNITIES FOR UTILITIES
In pursuit of a net zero scenario, several decarbonization strategies and numerous green technologies are under development. The best green technology can vary depending on process temperature, plant size and location, availability of feedstocks, technology readiness level (TRL), and level of efficiency. In addition to these technical parameters, market players must consider the impact of regulation and market incentives. Regulators can play a key role in accelerating maturity and economic competitiveness of a specific technology.
Table 1 provides a preliminary assessment of the main decarbonization technologies emerging in the market. Their measure of suitability is based on several factors, including industry reference temperature range, TRL, and economic competitiveness compared to alternative decarbonization technologies.
As shown in Table 1, a wide range of strategies are emerging to decarbonize industrial heat, including:
-
Direct electrification. This segment includes several technologies and offers valid decarbonization alternatives, such as:
-
Heat pumps — a mature technology with a high coefficient of performance (ranging from two to four) that fits well with low-temperature processes. It is found mainly in the food, beverage & tobacco industry and is potentially applicable for paper pulp, paper & graphic arts (but here the competition of biomass is fierce as the industry typically installs cogeneration plants). However, high retrofitting costs represent a barrier to technology diffusion, considering it requires several changes in production processes to substitute gas technologies (e.g., gas boilers).
-
Electric boilers — can reach higher temperatures than heat pumps (up to 300°C) and are applicable to several subindustries. The technology is already mature but is less efficient compared to other technologies, such as heat pumps. Although the maturity is quite high, further improvements in terms of scale and steam pressures are expected.
-
Ovens and dryers — mainly used in the food, beverage & tobacco industry for cooking (e.g., electric ovens), pasteurization and sterilization (e.g., microwave ovens), and drying (e.g., radiofrequency dryers) processes. The main difference between ovens and electric furnaces is that ovens can reach temperatures up to 500°C, while furnaces can be as high as 1,600°C. Even though they show good efficiency and high maturity, electric ovens and dryers are not more convenient than gas-fired ones. They will be preferred only in cases of very low electricity prices (self-production) and high natural gas costs.
-
Furnaces and kilns — can be applied in the main heat-demanding industries, although they face competition with hydrogen (e.g., ceramic) and biomass (e.g., concrete). They would be most suitable in the glass industry, where most of the heat produced by gas furnaces runs out of the system in the form of waste gas and dissipation, making electric furnaces a great competitor because they are more efficient and do not produce waste products. Electric glass-melting furnaces can thus be considered as economically attractive solutions. Furthermore, electric furnaces can be applied in combination with hydrogen within the iron & steel industry, which could potentially facilitate the development of this technology in this sector.
-
Power-to-heat (P2H) plus TES — could represent a key solution for medium-high temperature processes (up to 500°C), where current heat pump performances are less attractive. TES, fueled by electricity to generate and store heat that uses a heater to release it (P2H), allows ease of integration into production processes and modularity, making it a cross solution for use in many industries. Target industries include chemical & petrochemical; food, beverage & tobacco; and paper pulp, paper & graphic arts.
-
-
Indirect electrification. Hydrogen as a fuel makes sense for hard-to-abate industries where other electrification technologies are not applicable. For example, the iron and steel industry is looking closely at using hydrogen as an alternative to be used in two ways:
-
As an injection material instead of pulverized coal to improve the performance of furnaces. However, green hydrogen injection within these furnaces can guarantee reduction of a maximum of 20% of gas CO2 emissions.
-
As a reductant to gas to produce direct-reduced iron that then is processed with electric arc furnaces. The replacement of natural gas with hydrogen combined with the use of electric arc furnaces could ensure carbon-neutral steel production.
Note, however, that most current configurations are still not feasible without public incentives. The cost of producing, transporting, and storing hydrogen is currently higher than that for conventional fossil fuels, due to the immature technologies across the value chain and expensive long-haul transport. In addition, technology for producing and storing hydrogen is still developing, and it remains unclear how best to optimize processes for maximum efficiency. However, some circular models are emerging and, in general, hydrogen can be a competitor in high-temperature processes (e.g., iron and steel, ceramic).
-
CCUS. A technology used to capture carbon from industries (and power plants) for usage in products or permanent storage, CCUS can act as a bridge to the developing hydrogen economy, reducing short-term emissions of hard-to-abate industries, and providing a long-term solution in areas where alternative solutions (e.g., renewables, hydrogen) will not deliver effective decarbonization. The nonmetallic minerals (especially concrete and glass) and iron & steel industries are looking with interest to CCUS technology. The technology is of interest to industries that use CO2 within their production processes as well, such as the food, beverage & tobacco industry for the production of carbonated beverages and for protein synthesis.
-
Biomass. Biomass boilers (or biomass as fuel) are attractive technologies because of their low fuel price. However, these boilers are more expensive compared to electrification technologies, due to their complex fuel storage and handling systems. Feedstock availability is another barrier for biomass boiler use. However, biomass as a fuel is garnering the attention of two industries: paper pulp, paper & graphic arts, where it is spreading the use of black liquor to fuel cogeneration, and concrete, where the use of biomass as fuel in cement clinker kilns can reduce significantly their carbon intensity.
In this contest, TES is emerging as an attractive solution to decarbonize industrial heat consumption as it can be applied across many industries, it allows the integration of nonprogrammable renewable generation, and it is expected to become economically competitive in the short to medium term.
3
TES TECHNOLOGY
TES refers to the technology that allows for the storage and transfer of heat energy for later use, and it is fueled by electricity to generate heat. TES comprises a wide range of technology types, based on the processes/methods used to store and release heat, with two primary types:
-
Sensible — stores thermal energy by heating or cooling a storage medium (liquid or solid) without changing its phase
-
Latent — uses latent heat to store thermal energy, meaning the energy required to change the phase of the medium used
There are two additional types of TES on the market, but they are not yet finding industrial applications due to their installation complexity and low maturity, respectively: mechanical (compressed air energy storage and liquid air energy storage) and thermochemical (chemical looping, salt hydration, and absorption system).
Table 2 synthesizes eight main TES technologies of the sensible and latent types, reporting for each of them the temperature range covered and storage duration.
Depending on temperatures covered and storage duration, two of the most promising TES technologies for industrial applications belong to the sensible type and include:
-
Water tank TES — has the potential to become widely adopted due to its high technological maturity and suitability for industrial processes at low temperatures (up to 90°C). This technology collects heat in water reservoirs and uses it as an alternative heat source to replace hydrocarbon fuels.
-
Solid state — a key technology due to the wide temperature range it covers and its long storage duration. A fluid flowing through the material (primarily through metal pipes within it) transfers heat into the system during the charging phase and out of the system in the discharging phase. The technology has already achieved a high degree of maturity in temperatures up to 600°C-700°C, and further improvements are expected in the short to medium term to reach even higher temperatures.
In addition, molten salt is gaining industry attention, although industry applicability must be further assessed. With this technology, room-temperature solids (salts) are kept at temperatures above their melting point so that they are in liquid form and can reach higher temperatures (up to around 500°C).
HIGHLY FRAGMENTED TES VALUE CHAIN
The TES value chain is still under development. The vendor ecosystem is characterized by several small players engaged in pilot projects with industrial clients, while some large energy players are positioning themselves in the markets. Despite the highly fragmented nature of the market, vendors are investing mainly in sensible TES development, especially in solid-state technology, because it is more functional and adaptable to different types of industries. Figure 4 summarizes five current projects in the market.
WHY TES IS AN ATTRACTIVE SOLUTION
TES is part of a new wave of technologies that allows long-duration storage of energy, and it will play a key role in a future decarbonized energy world where energy will be produced by non-programmable renewable systems and stored to meet final energy demand.
In fact, renewable energy systems (RES) penetration has created a duck-shaped net electricity demand curve, which becomes more pronounced each year as solar capacity increases. While net demand is lowest at midday, demand in the evening or early morning increases as solar production significantly decreases. This results in demand exceeding available energy, leading to an imbalance between supply and demand, which, in turn, results in higher prices. The overproduction of solar energy can be exploited by charging TES during the day and discharging the stored thermal energy during evenings/early mornings.
A solution combining TES with a P2H technology is therefore a promising technology to decarbonize heat consumption up to 500°C. Given the temperatures and processes involved, the primary target industries are food, beverage & tobacco; chemical & petrochemical (especially pharmaceutical); and paper pulp, paper & graphic arts.
Levelized cost of heat (LCOH) analyses have demonstrated the economic competitiveness of an integrated photovoltaic plus TES solution compared to a gas boiler, even in the absence of incentive systems. We expect increasingly encouraging results, considering the favorable regulatory environment (governments are starting to establish dedicated funding programs to support TES deployment) and the anticipated reduction in technology costs due to the industrialization of production processes and R&D activities.
Overall, TES technology shows four main strengths that can be summarized as follows (see Figure 5):
-
Storage opportunities
-
Ease of installation
-
Modularity
-
Cost competitiveness
MAKING TES SOLUTION THRIVE
TES represents a promising technology for decarbonizing industrial heat consumption if widely adopted by industrial players. Three key enablers can make it happen:
-
Large-scale penetration of RES. With the increased deployment of RES, the technology will play a crucial role in balancing energy supply and demand by ensuring that energy can be stored at peak times and released at times of shortage to ensure constant industrial heat production. In addition, an increase in RES will lower energy prices, making electric technologies more competitive.
-
Government and policy maker support. Authorities should support technology deployment by promoting an ancillary service market, introducing mechanisms for lowering green electricity prices, and allocating public funds to make TES business cases profitable also in the short term.
-
Vendor scale-up. TES vendors should continue to invest in R&D to foster CAPEX reduction. New materials with more efficient storage capacity, improvements in thermal insulation, and improved designs can bring technology costs down in the short term. In addition, rising production volumes will increase the learning curve to further industrialize production processes. Private equity and funds should allocate capital to the most promising vendors to support the growth of the most valuable technology solutions.
4
INSIGHTS FOR UTILITIES
P2H plus TES technology has a vital role to play in successfully delivering a low-carbon economy. However, its ecosystem is still developing, and the winning technology is not yet clear. Several start-ups are emerging, and energy players are starting to assess the opportunity, but they are not clearly positioned along the value chain. To seize the opportunity of a technology that will play a crucial role in a rapidly growing market, utilities should focus on:
-
Technology and vendor intelligence. Although the technology has a good degree of maturity, utilities must continue to monitor the market through scouting activities to develop/consolidate their expertise on the technology and to capture breakthrough innovations, while also testing new vendors that enter the market. Utilities should submit requests for information to several vendors and launch pilot projects in the short term.
-
Commercial deployment. Utilities should define in detail the business models (e.g., spot sales) and partnership models (e.g., vendor remuneration schemes) they would like to adopt in order to launch commercial projects. This will allow them to fine-tune the models and to collect and leverage lessons learned to prepare the scale-up.
-
Advocacy. Leading players in the utility sector should play a role in advocating for investment in this technology. This would attract both public and private investments (e.g., private equity funds) and boost the growth of small operators. Using publications, events to educate and raise awareness, media involvement (e.g., press releases, interviews), and lobbying activities could ensure that the technology will quickly gain market share.
Barriers to industrial heat decarbonization are becoming anachronistic. On the one hand, the geopolitical situation and rising carbon prices make cheap fossil fuels less desirable. On the other hand, renewable energy systems are on the verge of a massive deployment, which would streamline access to green energy. Now is the time for utilities to step into the market to offer their industrial customers innovative solutions like P2H and TES.
Conclusion
Decarbonization of industrial heat consumption is a necessity as well as an opportunity for industries to embrace innovative solutions and contribute to a sustainable, low-carbon future. As the EU sets ambitious climate targets, it is crucial for key industries such as food, beverage & tobacco; chemical & petrochemical; and paper pulp, paper & graphic arts to adopt decarbonization strategies and technologies like P2H plus TES (direct electrification).
The potential of P2H plus TES technology lies in its ability to reduce CO2 emissions, integrate renewable energy systems, and offer economic competitiveness in the medium term. However, fully harnessing its potential will require support from governments, policy makers, and private investors. Utilities play a pivotal role in driving the adoption of these innovative solutions by continuously monitoring the market, defining business and partnership models, and advocating for public and private investments.
As the world moves toward a low-carbon economy, the barriers to industrial heat decarbonization are falling. With rising carbon prices and increasing accessibility to renewable energy sources, utilities must seize this opportunity to lead the transition toward a sustainable and low-carbon future. By offering innovative solutions for industrial heat decarbonization, utilities can make a lasting impact on their customers and the environment, showcasing a world where decarbonization is not only possible but also economically advantageous and environmentally responsible.
DOWNLOAD THE FULL REPORT
16 min read • Energy, Utilities & Resources
Decarbonizing industrial heat to face climate change
Is thermal energy storage the missing link in Europe’s industrial decarbonization strategy?
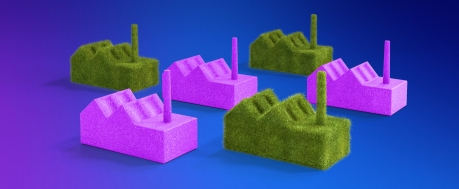
DATE
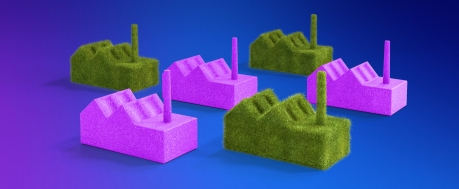
Executive Summary
Decarbonizing industrial heat consumption is crucial to reduce carbon emissions and mitigate the effects of climate change. Currently, the top five heat-demanding industries in the EU — chemical & petrochemical; nonmetallic minerals; food, beverage & tobacco; paper pulp, paper & graphic arts; and iron & steel — account for more than 80% of heat consumption and urgently need decarbonization strategies.
Direct and indirect electrification, carbon capture, utilization, and storage (CCUS), and biomass are among the main technologies for decarbonizing industrial heat. Thermal energy storage (TES) is a potential solution for direct electrification, particularly for industries with process temperatures that reach up to 500°C. Key strengths of TES technology include storage opportunities (i.e., ability to store heat for later use and to integrate non-programmable renewable systems such as photovoltaics and wind), ease of installation, modularity, and cost competitiveness.
Utilities can seize the opportunity and be pioneers in this market by focusing on testing the technology and building partnerships with vendors to speed up commercial deployment. Additionally, they can implement advocacy strategies with European and local authorities.
Innovative solutions like TES can help industrial customers achieve their decarbonization goals, contributing to a low-carbon economy.
1
EUROPEAN INDUSTRIAL HEAT CONSUMPTION
DECARBONIZATION AS A MUST-HAVE
Regulators in Europe are accelerating the transition toward a low-carbon economy, pushing the industrial sector to reduce the CO2 footprint. The EU has set ambitious targets to reduce greenhouse gas emissions by at least 55% by 2030 and achieve carbon neutrality by 2050. However, despite renewable expansion and the increasing attention on the hydrogen economy, these targets are projected to be missed. New strategies are needed to achieve climate targets, and decarbonization of industrial heat consumption through innovative technologies will play a pivotal role.
Industrial heat consumption accounts for a significant portion of global energy usage, with two-thirds of industrial energy demand and almost a fifth of global energy consumption attributed to it, according to the International Energy Agency (IEA). Most industrial heat is generated through the combustion of natural gas, fossil fuels, and petrol, resulting in the emission of substantial amounts of CO2. Thus, decarbonizing industrial heat consumption is essential to reduce carbon emissions.
EUROPEAN ADDRESSABLE MARKET
In Europe, decarbonization of industrial heat consumption offers an opportunity for energy players and vendors to develop innovative solutions to replace non-green fuels and to jump into a market that will face a boost in the coming years.
According to Eurostat, the European industrial sector consumes ~2,500 terawatts per hour (TWh) of energy from different sources (see Figure 1), of which ~1,800 TWh are used to produce heat (accounting for 72% of the market). The share of natural gas, petrol, and fossil fuels used to produce heat is around 60% of the total, generating an addressable market for decarbonization of ~1,100 TWh.
TOP 5 HEAT-DEMANDING INDUSTRIES
Industrial heat consumption is highly concentrated in five industries, which comprise more than 80% of consumption: chemical & petrochemical; nonmetallic minerals; food, beverage & tobacco; paper pulp, paper & graphic arts; and iron & steel. These industries use fuels such as natural gas, fossil fuel, and petrol for heat generation. As Figure 2 illustrates, the heat to be decarbonized often exceeds 60% of the total sources of fuel used for heat generation. Together, these industries show an addressable market (i.e., the total amount of natural gas, fossil fuels, and petrol used to produce heat to be decarbonized) equal to ~930 TWh.
INDUSTRY OPPORTUNITIES
The addressable market of these top five industries can also be examined according to temperature, as shown in Figure 3, revealing substantial differences among them and demonstrating that some industries are more suitable than others for decarbonization (e.g., industries with lower-temperature production processes are significantly more suitable for decarbonization than those using higher temperatures). In temperatures exceeding 500°C, competition of natural gas, fossil fuels, and petrol are very high and electrification technologies are still under development.
Analyzing the production processes of the top five industries in terms of heat demand reveals the following about their decarbonization potential:
-
Chemical & petrochemical — temperatures concentrated in the range of 100°C-500°C. The pharmaceutical subindustry represents a huge opportunity for operators trying to push decarbonization due to its low temperatures (not exceeding 250°C), suitability of the processes, and use of technology that can be easily replaced (e.g., gas boilers, which are used mainly in granulation and melting phases).
-
Nonmetallic minerals — very high temperatures (often more than 1,000°C). Three main subindustries include:
-
Concrete — temperatures reaching 1,500°C (clinker-manufacturing phase), for which furnaces fueled by gas/oil are widespread. However, the use of biomass and hydrogen are gaining attention.
-
Ceramic — temperatures reaching 1,200°C (firing phase) through the use of tunnel kilns fueled by gas/oil. There is a strong interest in hydrogen for this industry. Electrification in the short term could face barriers, but green technologies are possible for the drying phase (up to 200°C).
-
Glass — temperatures ranging from 500°C (surface-heating phase) to 800°C (melting phase), leaving more room for decarbonization compared to concrete and ceramic. This subindustry mainly uses gas ovens. Within the nonmetallic mineral segment, glass may have the greatest potential for decarbonization, as electric ovens already have a high degree of maturity.
-
Food, beverage & tobacco — temperatures mainly below 200°C. In this industry, meat and milk and dairy have the most potential in terms of size and suitability of the processes. Cooking (80°C-120°C) and pasteurization (60°C-70°C) phases can be easily electrified (currently using mainly gas boilers and plate heat exchangers, respectively). Technologies such as heat pumps are already gaining market share.
-
Iron & steel — very high temperatures (mainly more than 1,000°C) for all process phases (i.e., sintering, melting, and rolling). Industry players have widely adopted gas furnaces. However, electrification technologies (e.g., electric arc furnaces) are possible, including hybrid configurations that involve the use of hydrogen. Huge CAPEX, retrofitting costs, and high electricity prices are the main barriers for electrification within the industry. Strong political commitment from regulators in providing incentives and subsidies can be a boost for electrification in the short term.
-
Paper pulp, paper & graphic arts — temperatures mainly below 200°C. Industry is well suited to electrification due to low temperatures in all process phases (i.e., pulping, washing and bleaching, pressing and drying, and calendering) and technologies that exist already in the market. However, this industry is characterized by extensive use of cogenerations fueled by gas, for which major industry players invested a lot of capital. Furthermore, the industry is investigating the use of black liquor (a natural byproduct obtained from paper processing) to feed cogenerations as an alternative to gas and a complementary strategy to decarbonize heat consumption. Electrification is possible in the short to medium term, but considerable barriers (cogenerations) and threats (use of black liquor) exist.
To summarize, utilities should focus first on three main industries to push decarbonization of industrial heat consumption: food, beverage & tobacco; chemical & petrochemical; and paper pulp, paper & graphic arts.
2
DECARBONIZATION STRATEGIES
OPPORTUNITIES FOR UTILITIES
In pursuit of a net zero scenario, several decarbonization strategies and numerous green technologies are under development. The best green technology can vary depending on process temperature, plant size and location, availability of feedstocks, technology readiness level (TRL), and level of efficiency. In addition to these technical parameters, market players must consider the impact of regulation and market incentives. Regulators can play a key role in accelerating maturity and economic competitiveness of a specific technology.
Table 1 provides a preliminary assessment of the main decarbonization technologies emerging in the market. Their measure of suitability is based on several factors, including industry reference temperature range, TRL, and economic competitiveness compared to alternative decarbonization technologies.
As shown in Table 1, a wide range of strategies are emerging to decarbonize industrial heat, including:
-
Direct electrification. This segment includes several technologies and offers valid decarbonization alternatives, such as:
-
Heat pumps — a mature technology with a high coefficient of performance (ranging from two to four) that fits well with low-temperature processes. It is found mainly in the food, beverage & tobacco industry and is potentially applicable for paper pulp, paper & graphic arts (but here the competition of biomass is fierce as the industry typically installs cogeneration plants). However, high retrofitting costs represent a barrier to technology diffusion, considering it requires several changes in production processes to substitute gas technologies (e.g., gas boilers).
-
Electric boilers — can reach higher temperatures than heat pumps (up to 300°C) and are applicable to several subindustries. The technology is already mature but is less efficient compared to other technologies, such as heat pumps. Although the maturity is quite high, further improvements in terms of scale and steam pressures are expected.
-
Ovens and dryers — mainly used in the food, beverage & tobacco industry for cooking (e.g., electric ovens), pasteurization and sterilization (e.g., microwave ovens), and drying (e.g., radiofrequency dryers) processes. The main difference between ovens and electric furnaces is that ovens can reach temperatures up to 500°C, while furnaces can be as high as 1,600°C. Even though they show good efficiency and high maturity, electric ovens and dryers are not more convenient than gas-fired ones. They will be preferred only in cases of very low electricity prices (self-production) and high natural gas costs.
-
Furnaces and kilns — can be applied in the main heat-demanding industries, although they face competition with hydrogen (e.g., ceramic) and biomass (e.g., concrete). They would be most suitable in the glass industry, where most of the heat produced by gas furnaces runs out of the system in the form of waste gas and dissipation, making electric furnaces a great competitor because they are more efficient and do not produce waste products. Electric glass-melting furnaces can thus be considered as economically attractive solutions. Furthermore, electric furnaces can be applied in combination with hydrogen within the iron & steel industry, which could potentially facilitate the development of this technology in this sector.
-
Power-to-heat (P2H) plus TES — could represent a key solution for medium-high temperature processes (up to 500°C), where current heat pump performances are less attractive. TES, fueled by electricity to generate and store heat that uses a heater to release it (P2H), allows ease of integration into production processes and modularity, making it a cross solution for use in many industries. Target industries include chemical & petrochemical; food, beverage & tobacco; and paper pulp, paper & graphic arts.
-
-
Indirect electrification. Hydrogen as a fuel makes sense for hard-to-abate industries where other electrification technologies are not applicable. For example, the iron and steel industry is looking closely at using hydrogen as an alternative to be used in two ways:
-
As an injection material instead of pulverized coal to improve the performance of furnaces. However, green hydrogen injection within these furnaces can guarantee reduction of a maximum of 20% of gas CO2 emissions.
-
As a reductant to gas to produce direct-reduced iron that then is processed with electric arc furnaces. The replacement of natural gas with hydrogen combined with the use of electric arc furnaces could ensure carbon-neutral steel production.
Note, however, that most current configurations are still not feasible without public incentives. The cost of producing, transporting, and storing hydrogen is currently higher than that for conventional fossil fuels, due to the immature technologies across the value chain and expensive long-haul transport. In addition, technology for producing and storing hydrogen is still developing, and it remains unclear how best to optimize processes for maximum efficiency. However, some circular models are emerging and, in general, hydrogen can be a competitor in high-temperature processes (e.g., iron and steel, ceramic).
-
CCUS. A technology used to capture carbon from industries (and power plants) for usage in products or permanent storage, CCUS can act as a bridge to the developing hydrogen economy, reducing short-term emissions of hard-to-abate industries, and providing a long-term solution in areas where alternative solutions (e.g., renewables, hydrogen) will not deliver effective decarbonization. The nonmetallic minerals (especially concrete and glass) and iron & steel industries are looking with interest to CCUS technology. The technology is of interest to industries that use CO2 within their production processes as well, such as the food, beverage & tobacco industry for the production of carbonated beverages and for protein synthesis.
-
Biomass. Biomass boilers (or biomass as fuel) are attractive technologies because of their low fuel price. However, these boilers are more expensive compared to electrification technologies, due to their complex fuel storage and handling systems. Feedstock availability is another barrier for biomass boiler use. However, biomass as a fuel is garnering the attention of two industries: paper pulp, paper & graphic arts, where it is spreading the use of black liquor to fuel cogeneration, and concrete, where the use of biomass as fuel in cement clinker kilns can reduce significantly their carbon intensity.
In this contest, TES is emerging as an attractive solution to decarbonize industrial heat consumption as it can be applied across many industries, it allows the integration of nonprogrammable renewable generation, and it is expected to become economically competitive in the short to medium term.
3
TES TECHNOLOGY
TES refers to the technology that allows for the storage and transfer of heat energy for later use, and it is fueled by electricity to generate heat. TES comprises a wide range of technology types, based on the processes/methods used to store and release heat, with two primary types:
-
Sensible — stores thermal energy by heating or cooling a storage medium (liquid or solid) without changing its phase
-
Latent — uses latent heat to store thermal energy, meaning the energy required to change the phase of the medium used
There are two additional types of TES on the market, but they are not yet finding industrial applications due to their installation complexity and low maturity, respectively: mechanical (compressed air energy storage and liquid air energy storage) and thermochemical (chemical looping, salt hydration, and absorption system).
Table 2 synthesizes eight main TES technologies of the sensible and latent types, reporting for each of them the temperature range covered and storage duration.
Depending on temperatures covered and storage duration, two of the most promising TES technologies for industrial applications belong to the sensible type and include:
-
Water tank TES — has the potential to become widely adopted due to its high technological maturity and suitability for industrial processes at low temperatures (up to 90°C). This technology collects heat in water reservoirs and uses it as an alternative heat source to replace hydrocarbon fuels.
-
Solid state — a key technology due to the wide temperature range it covers and its long storage duration. A fluid flowing through the material (primarily through metal pipes within it) transfers heat into the system during the charging phase and out of the system in the discharging phase. The technology has already achieved a high degree of maturity in temperatures up to 600°C-700°C, and further improvements are expected in the short to medium term to reach even higher temperatures.
In addition, molten salt is gaining industry attention, although industry applicability must be further assessed. With this technology, room-temperature solids (salts) are kept at temperatures above their melting point so that they are in liquid form and can reach higher temperatures (up to around 500°C).
HIGHLY FRAGMENTED TES VALUE CHAIN
The TES value chain is still under development. The vendor ecosystem is characterized by several small players engaged in pilot projects with industrial clients, while some large energy players are positioning themselves in the markets. Despite the highly fragmented nature of the market, vendors are investing mainly in sensible TES development, especially in solid-state technology, because it is more functional and adaptable to different types of industries. Figure 4 summarizes five current projects in the market.
WHY TES IS AN ATTRACTIVE SOLUTION
TES is part of a new wave of technologies that allows long-duration storage of energy, and it will play a key role in a future decarbonized energy world where energy will be produced by non-programmable renewable systems and stored to meet final energy demand.
In fact, renewable energy systems (RES) penetration has created a duck-shaped net electricity demand curve, which becomes more pronounced each year as solar capacity increases. While net demand is lowest at midday, demand in the evening or early morning increases as solar production significantly decreases. This results in demand exceeding available energy, leading to an imbalance between supply and demand, which, in turn, results in higher prices. The overproduction of solar energy can be exploited by charging TES during the day and discharging the stored thermal energy during evenings/early mornings.
A solution combining TES with a P2H technology is therefore a promising technology to decarbonize heat consumption up to 500°C. Given the temperatures and processes involved, the primary target industries are food, beverage & tobacco; chemical & petrochemical (especially pharmaceutical); and paper pulp, paper & graphic arts.
Levelized cost of heat (LCOH) analyses have demonstrated the economic competitiveness of an integrated photovoltaic plus TES solution compared to a gas boiler, even in the absence of incentive systems. We expect increasingly encouraging results, considering the favorable regulatory environment (governments are starting to establish dedicated funding programs to support TES deployment) and the anticipated reduction in technology costs due to the industrialization of production processes and R&D activities.
Overall, TES technology shows four main strengths that can be summarized as follows (see Figure 5):
-
Storage opportunities
-
Ease of installation
-
Modularity
-
Cost competitiveness
MAKING TES SOLUTION THRIVE
TES represents a promising technology for decarbonizing industrial heat consumption if widely adopted by industrial players. Three key enablers can make it happen:
-
Large-scale penetration of RES. With the increased deployment of RES, the technology will play a crucial role in balancing energy supply and demand by ensuring that energy can be stored at peak times and released at times of shortage to ensure constant industrial heat production. In addition, an increase in RES will lower energy prices, making electric technologies more competitive.
-
Government and policy maker support. Authorities should support technology deployment by promoting an ancillary service market, introducing mechanisms for lowering green electricity prices, and allocating public funds to make TES business cases profitable also in the short term.
-
Vendor scale-up. TES vendors should continue to invest in R&D to foster CAPEX reduction. New materials with more efficient storage capacity, improvements in thermal insulation, and improved designs can bring technology costs down in the short term. In addition, rising production volumes will increase the learning curve to further industrialize production processes. Private equity and funds should allocate capital to the most promising vendors to support the growth of the most valuable technology solutions.
4
INSIGHTS FOR UTILITIES
P2H plus TES technology has a vital role to play in successfully delivering a low-carbon economy. However, its ecosystem is still developing, and the winning technology is not yet clear. Several start-ups are emerging, and energy players are starting to assess the opportunity, but they are not clearly positioned along the value chain. To seize the opportunity of a technology that will play a crucial role in a rapidly growing market, utilities should focus on:
-
Technology and vendor intelligence. Although the technology has a good degree of maturity, utilities must continue to monitor the market through scouting activities to develop/consolidate their expertise on the technology and to capture breakthrough innovations, while also testing new vendors that enter the market. Utilities should submit requests for information to several vendors and launch pilot projects in the short term.
-
Commercial deployment. Utilities should define in detail the business models (e.g., spot sales) and partnership models (e.g., vendor remuneration schemes) they would like to adopt in order to launch commercial projects. This will allow them to fine-tune the models and to collect and leverage lessons learned to prepare the scale-up.
-
Advocacy. Leading players in the utility sector should play a role in advocating for investment in this technology. This would attract both public and private investments (e.g., private equity funds) and boost the growth of small operators. Using publications, events to educate and raise awareness, media involvement (e.g., press releases, interviews), and lobbying activities could ensure that the technology will quickly gain market share.
Barriers to industrial heat decarbonization are becoming anachronistic. On the one hand, the geopolitical situation and rising carbon prices make cheap fossil fuels less desirable. On the other hand, renewable energy systems are on the verge of a massive deployment, which would streamline access to green energy. Now is the time for utilities to step into the market to offer their industrial customers innovative solutions like P2H and TES.
Conclusion
Decarbonization of industrial heat consumption is a necessity as well as an opportunity for industries to embrace innovative solutions and contribute to a sustainable, low-carbon future. As the EU sets ambitious climate targets, it is crucial for key industries such as food, beverage & tobacco; chemical & petrochemical; and paper pulp, paper & graphic arts to adopt decarbonization strategies and technologies like P2H plus TES (direct electrification).
The potential of P2H plus TES technology lies in its ability to reduce CO2 emissions, integrate renewable energy systems, and offer economic competitiveness in the medium term. However, fully harnessing its potential will require support from governments, policy makers, and private investors. Utilities play a pivotal role in driving the adoption of these innovative solutions by continuously monitoring the market, defining business and partnership models, and advocating for public and private investments.
As the world moves toward a low-carbon economy, the barriers to industrial heat decarbonization are falling. With rising carbon prices and increasing accessibility to renewable energy sources, utilities must seize this opportunity to lead the transition toward a sustainable and low-carbon future. By offering innovative solutions for industrial heat decarbonization, utilities can make a lasting impact on their customers and the environment, showcasing a world where decarbonization is not only possible but also economically advantageous and environmentally responsible.
DOWNLOAD THE FULL REPORT